
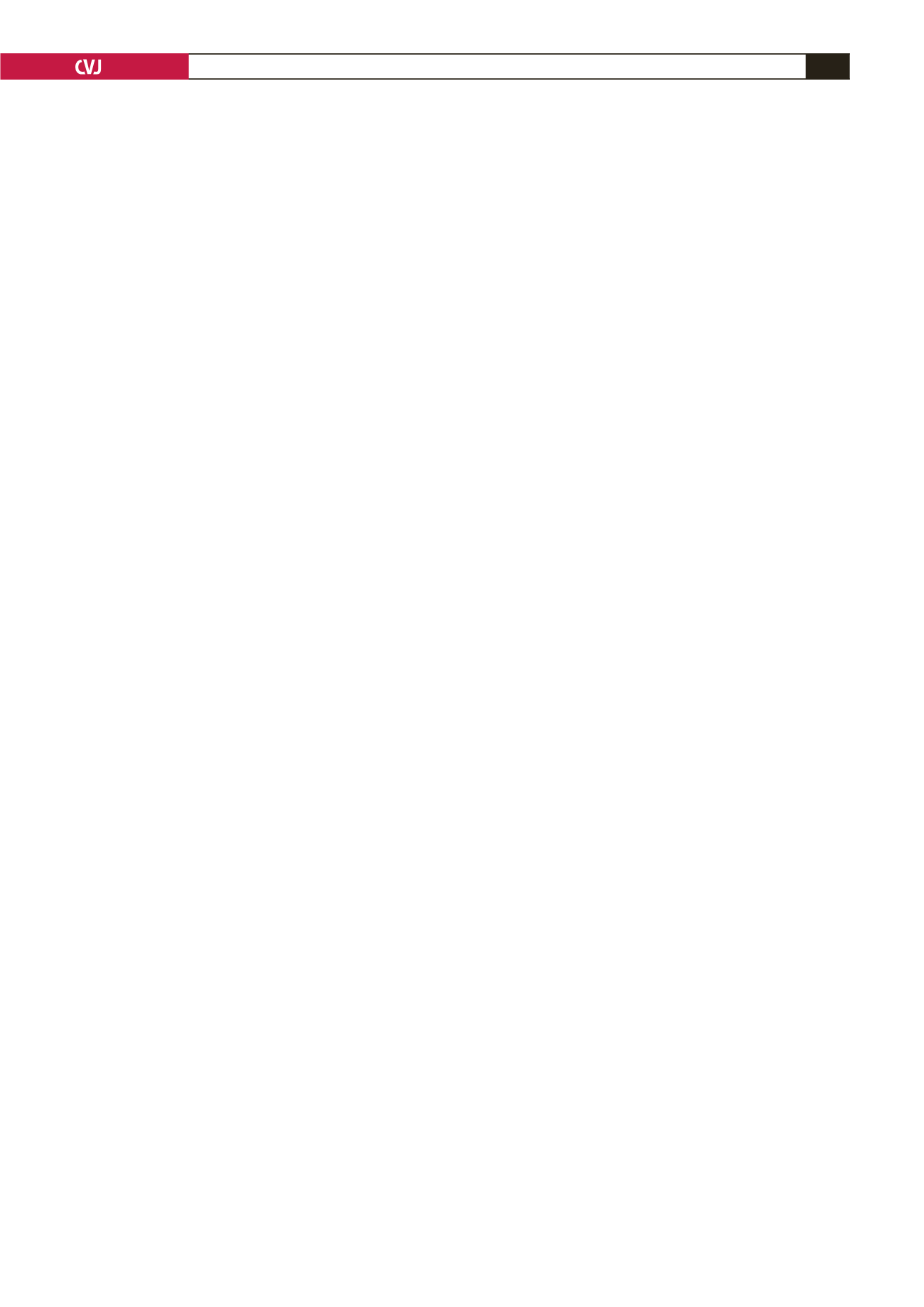
CARDIOVASCULAR JOURNAL OF AFRICA • Volume 30, No 5, September/October 2019
AFRICA
273
contraction. In our study, it was observed that
I
Na
had first been
increased and then decreased in the simulated ischaemic state.
Therefore, in the early stage of ischaemia, cardiomyocytes were
in a heterogeneous ischaemic state, and the dispersion of
I
Na
in
ischaemic tissue would be increased with the prolongation of
ischaemia, which may be one of the bases for the formation of
local abnormal current.
As a basic drug of acute coronary syndrome, statins have
been shown to reduce the morbidity of ventricular arrhythmias
and the mortality rate.
18,19
Therefore we observed the effect of
atorvastatin on
I
Na
, which was in the early stage of ischaemia,
and found that the increased current was inhibited. As we
know, before producing pleiotropic effects, statins should inhibit
HMG-CoA reductase and then block the important mevalonate
pathway.
20,21
However, Gerber
et al
. showed that atorvastatin
decreased the HMG-CoA reductase activity in L cells only after
incubation with the drug for 18 hours.
22
In addition, Vaquero
et
al
. demonstrated the membrane capacitance was not changed
by atorvastatin.
11
Therefore, non-specific perturbation of the
membrane seems a very unlikely mechanism for atorvastatin to
be responsible for, otherwise the capacitance would be changed
as the dielectric constant had been modified.
As a fat-soluble statin, atorvastatin calcium is slightly soluble
in pH 7.4 phosphate buffer, which means that the theoretical
maximum range of atorvastatin is 82.68 to 826.8 μmol/l. We used
a concentration of 5 μmol/l, which was equivalent to the clinical
dose of 20–80 mg/d.
23
This could avoid the use of a fat-soluble
solvent, which may also influence the membrane currents.
Conclusions
In this study we observed the time-dependent effect of atorvastatin
on
I
Na
in a simulated ischaemic condition and found that the
phenomenon of transiently increased
I
Na
disappeared. The gated
characteristics showed that atorvastatin reduced
K
i
and weakened
the decline of
τ
value caused by ischaemia. Therefore the channel
inactivation was faster and the recovery was slower, which caused
the number of open channels per unit time to decrease, finally
resulting in a decrease in whole-cell current.
Atorvastatin inhibited the abnormal increase of
I
Na
during
the early stage of simulated ischaemia by acting on the processes
of inactivation and recovery. As statins can block the activity
of a voltage-gated calcium channel,
24
atorvastatin could also
transiently block the sodium channel when entering the cell
during the first three to seven minutes of ischaemia. Interestingly,
atorvastatin appeared to prevent a further decrease in
I
Na
as the
ischaemic time extended to more than 19 minutes, indicating
another cardioprotective effect of atorvastatin, in preventing
further ischaemic injury (such as ischaemic postconditioning of
statins
25
). Therefore atorvastatin played a role only as a buffer in
abating rapid changes in
I
Na
over time during early ischaemia,
which helped to reduce the electrical heterogeneity of the
ischaemic myocardium
26,27
and improve the cardiac arrhythmia
matrix effect.
References
1.
Glinge C, Sattler S, Jabbari R, Tfelt-Hansen J. Epidemiology and
genetics of ventricular fibrillation during acute myocardial infarction.
J
Geriatr Cardiol
2016;
13
(9): 789–797.
2.
Henkel DM, Witt BJ, Gersh BJ, Jacobsen SJ, Weston SA, Meverden
RA,
et al.
Ventricular arrhythmias after acute myocardial infarction: a
20-year community study.
Am Heart J
2006;
151
(4): 806–812.
3.
Veerman CC, Wilde AA, Lodder EM. The cardiac sodium channel gene
SCN5A and its gene product NaV1.5: Role in physiology and patho-
physiology.
Gene
2015;
573
(2): 177–187.
4.
Zhao Z, Yin Y, Wu H, Jiang M, Lou J, Bai G,
et al.
Arctigenin, a
potential anti-arrhythmic agent, inhibits aconitine-induced arrhythmia
by regulating multi-ion channels.
Cell Physiol Biochem
2013;
32
(5):
1342–1353.
5.
Ding C, Fu XH, He ZS, Chen HX, Xue L, Li JX. Cardioprotective
effects of simvastatin on reversing electrical remodeling induced by
myocardial ischemia–reperfusion in normocholesterolemic rabbits.
Chin
Med J
(Engl) 2008;
121
(6): 551–556.
6.
Wei X, Zhu A, Zhang Y, Yao S, Mao W. Pre- and delayed treatments
with ranolazine ameliorate ventricular arrhythmias and Nav1.5 down-
regulation in ischemic/reperfused rat hearts.
J Cardiovasc Pharmacol
2016;
68
(4): 269–279.
7.
Das MK, Zipes DP. Antiarrhythmic and nonantiarrhythmic drugs for
sudden cardiac death prevention.
J Cardiovasc Pharmacol
2010;
55
(5):
438–449.
8.
Apiyasawat S, Sritara P, Ngarmukos T, Sriratanasathavorn C,
Kasemsuwan P. Association of statin therapy with ventricular arrhyth-
mias among patients with acute coronary syndrome.
Heart Asia
2013;
5
(1): 39–41.
9.
Al-Khatib SM, Stevenson WG, Ackerman MJ, Bryant WJ, Callans
DJ, Curtis AB,
et al
. 2017 AHA/ACC/HRS guideline for manage-
ment of patients with ventricular arrhythmias and the prevention of
sudden cardiac death: A report of the American College of Cardiology/
American Heart Association task force on clinical practice guidelines
and the Heart Rhythm Society.
Circulation
2018;
138
: e272–e391.
10. Oesterle A, Laufs U, Liao JK. Pleiotropic effects of statins on the
cardiovascular system.
Circ Res
2017;
120
(1): 229–243.
11. Vaquero M, Caballero R, Gomez R, Nunez L, Tamargo J, Delpon E.
Effects of atorvastatin and simvastatin on atrial plateau currents.
J Mol
Cell Cardiol
2007;
42
(5): 931–945.
12. Opitz CF, Mitchell GF, Pfeffer MA, Pfeffer JM. Arrhythmias and
death after coronary artery occlusion in the rat. Continuous telemetric
ECG monitoring in conscious, untethered rats.
Circulation
1995;
92
(2):
253–261.
13. Karmazinova M, Lacinova L. Measurement of cellular excitability by
whole cell patch clamp technique.
Physiol Res
2010;
59
(Suppl 1): S1–7.
14. Carmeliet E. Cardiac ionic currents and acute ischemia: from channels
to arrhythmias.
Physiol Rev
1999;
79
(3): 917–1017.
15. Huang CL. Murine electrophysiological models of cardiac arrhythmo-
genesis.
Physiol Rev
2017;
97
(1): 283–409.
16. Chan TY. Aconite poisoning.
Clin Toxicol
(Phila) 2009;
47
(4): 279–285.
17. Coulson JM, Caparrotta TM, Thompson JP. The management of
ventricular dysrhythmia in aconite poisoning.
Clin Toxicol
(Phila) 2017;
55
(5): 313–321.
18. Beri A, Contractor T, Gardiner JC, Ardhanari S, Thakur R. Reduction
in the intensity rate of appropriate shocks for ventricular arrhythmias
with statin therapy.
J Cardiovasc Pharmacol
2010;
56
(2): 190–194.
19. Chung CM, Lin MS, Chang CH, Cheng HW, Chang ST, Wang PC,
et al
. Moderate to high intensity statin in dialysis patients after acute
myocardial infarction: A national cohort study in Asia.
Atherosclerosis
2017;
267
: 158–166.
20. Margaritis M, Sanna F, Antoniades C. Statins and oxidative stress in
the cardiovascular system.
Curr Pharm Des
2017 Sep 26. [Epub ahead
of print].