
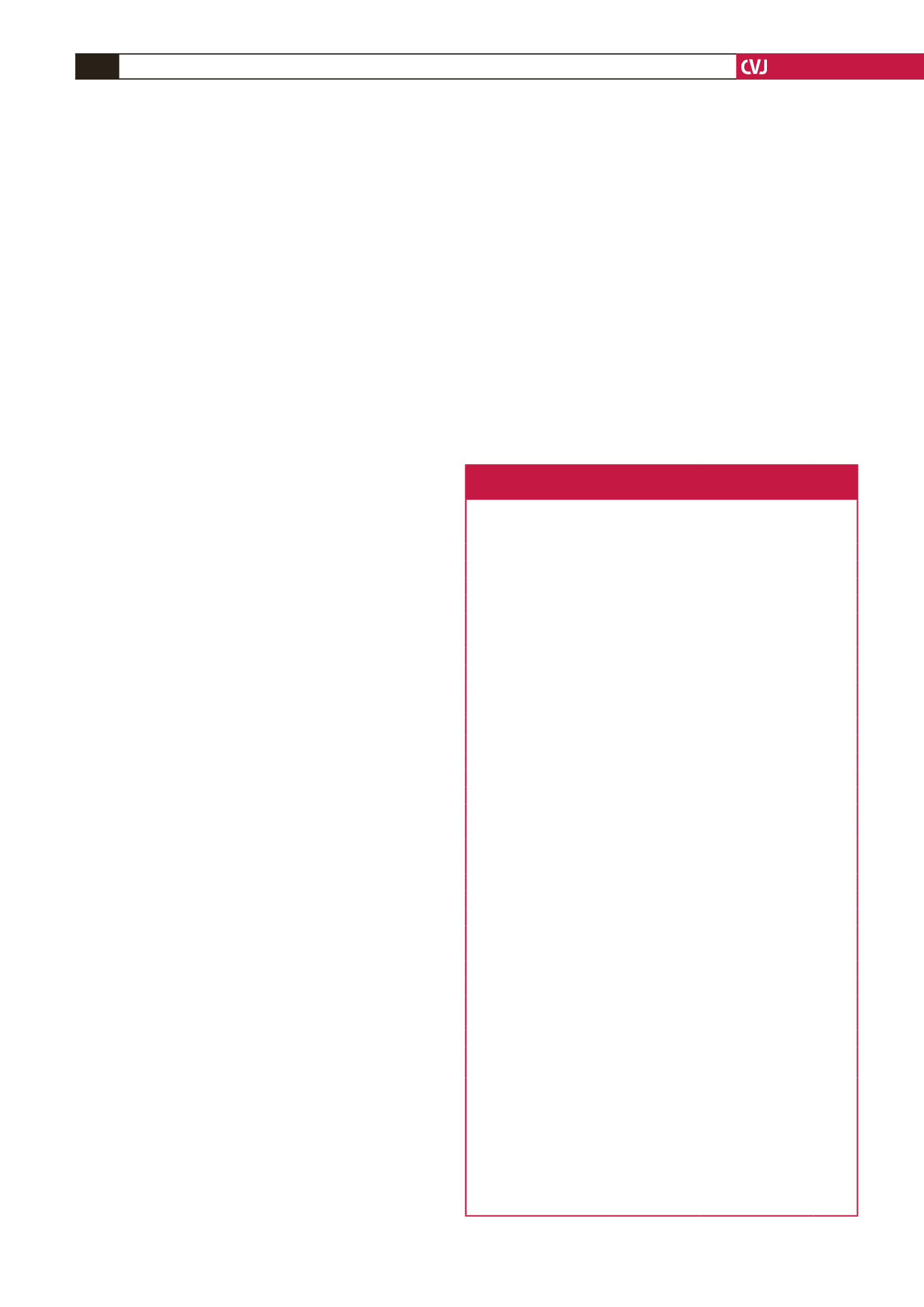
CARDIOVASCULAR JOURNAL OF AFRICA • Volume 28, No 2, March/April 2017
128
AFRICA
subsequently activates the oxidative stress/inflammation cascade.
This in turn underlies insulin resistance and the evolution of
micro- and macrovascular complications (Fig. 1, pathways:
3a-53-blood glucose-54-PI3K:MAPK-69-insulin resistance-72-
ROS). Hyperinsulinaemia, by itself, contributes significantly to
atherogenecity, leading to CHD.
12
An increase in plasma free fatty acid (FFA) concentrations
plays a key role in the pathogenesis of insulin resistance through
actions that block insulin signal transduction. An increase in FFA
levels results in induction of oxidative stress, low-grade systemic
inflammation, and subnormal vascular reactivity, in addition
to causing insulin resistance.
5
As insulin resistance also results
in the relative non-suppression of adipocyte hormone-sensitive
lipase,
13
there is further enhancement in lipolysis, increased FFA
and insulin resistance. As insulin suppresses pro-inflammatory
transcription factors, such as nuclear factor-
κβ
(NF-
κβ
), and
also suppresses reactive oxygen species (ROS) generation, insulin
resistance therefore also has a comprehensive pro-inflammatory
effect (Fig. 1, pathways: 3c-18-FFA-37-plasma lipids-34-12-
LDL-33-oxLDL-51-hypercholesterolaemia).
Fig. 1 therefore shows why an insulin-resistant state may be
pro-inflammatory. The origin of the insulin resistance may be
traced back to the pro-inflammatory cytokine TNF-
α
, which
is expressed by adipose tissue.
14
Adipose tissue has been shown
to express not only TNF-
α
, but also other pro-inflammatory
mediators, including CRP. Macrophages residing in the adipose
tissue may also be a source of pro-inflammatory factors and they
can also modulate the secretory activities of adipocytes
15
(Fig. 1,
pathway: 3c-21-TNF
α
/IL6).
During regular moderate exercise, IL-6 is produced by skeletal
muscle fibres via a TNF-independent pathway. IL-6 stimulates
the appearance in the circulation of anti-inflammatory cytokines,
which inhibit the production of pro-inflammatory TNF-
α
.
16
Additionally, IL-6 enhances lipid turnover, stimulating lipolysis
as well as fat oxidation. Regular physical exercise therefore
induces suppression of TNF-
α
and thereby offers protection
against TNF-
α
-induced insulin resistance.
16
Low-grade systemic
inflammation therefore appears to be aetiologically linked to
the pathogenesis of CHD,
17
countered by moderate exercise
with its anti-inflammatory effects
5
(Fig. 1, pathway: 3a-53-blood
glucose-54-69-insulin resistance-71).
The adipokine adiponectin is anti-inflammatory and
potentially anti-atherogenic.
5
Low adiponectin levels act as a
marker for CHD and are associated with overweight subjects.
18
Regular physical exercise (and an energy-controlled diet) reduces
visceral fat mass, with a subsequent increased release of anti-
inflammatory adiponectin, therefore resulting in reduced risk of
CHD
19
(Fig. 1, pathway: 3c-19-39-insulin resistance).
Lack of physical exercise may lead to hypertension, another
CHD hallmark, through increased vascular and sympathetic
tone created by reduced bioavailability of nitrous oxide (NO) and
activation of the renin–angiotensin system
20,
21
(Fig. 1, pathway:
3a-53-blood glucose-54-60-72-vasodilation). Hypertension is
directly correlated with visceral fat mass, which may be decreased
by moderate exercise.
22
The lower blood glucose levels that result from moderate
exercise lead to a reduction in the phosphatidylinositol 3-kinase
(PI3K) to mitogen-activated protein kinase (MAPK) ratio,
which in turn decreases insulin resistance
23
(Fig. 1, pathway:
3a-53-blood glucose-54-69-72-73-hypercoagulabilty). Increased
insulin sensitivity decreases serum levels of platelet factors and
thus reduces the potential for hypercoagulability.
24,25
Moderate exercise also increases coronary blood flow,
26
which
increases the release of prostaglandins.
27
This is important in
heart microvasculature, in which prostaglandins are substantially
involved in flow-mediated vasodilation.
27
Moderate exercise acts on the central nervous system by
decreasing serum cortisol levels.
28
This in turn reduces insulin
resistance, which decreases angiotensin II levels and results
in reduced hypertension. Reactive oxygen species (ROS) and
cyclooxygenase (COX) 1/2 levels reduce concomitantly, which
lead to a lower inflammatory state
20
(Fig. 1, pathway: insulin
resistance-85-inflammatory state).
It is apparent that moderate exercise directly and indirectly
affects a plethora of interconnected pathogenetic mechanisms.
Each CHD hallmark and pathogenetic trait can amplify the
Table 2. Association between biomarkers and
prediction of CHD relative risk
Biomarker
(class and salient examples)
Prediction of
CHD relative
risk (95% CI)
Size of studies
(N
=
number of trials,
n
=
number of patients)
Refer-
ences
Lipid-related markers
Triglycerides
0.99 (0.94–1.05) (
N
=
68,
n
=
302 430)
63
LDL
1.25 (1.18–1.33) (
N
=
15,
n
=
233 455)
64
HDL
0.78 (0.74–0.82) (
N
=
68,
n
=
302 430)
63
Apo B
1.43 (1.35–1.51) (
N
=
15,
n
=
233 455)
64
Leptin
1.04 (0.92–1.17)
(
n
=
1 832)
65
Inflammatory markers
hsCRP
1.20 (1.18–1.22) (
N
=
38,
n
=
166 596)
66
IL-6
1.25 (1.19–1.32) (
N
=
25,
n
=
42 123)
67
TNF-
α
1.17 (1.09–1.25)
(
N
=
7,
n
=
6 107)
67
GDF-15
1.40 (1.10–1.80)
(
n
=
1 740)
68
OPG
1.41 (1.33–1.57)
(
n
=
5 863)
69
Marker of oxidative stress
MPO
1.17 (1.06–1.30)
(
n
=
2 861)
70
Marker of vascular function and neurohormonal activity
BNP
1.42 (1.24–1.63) (
N
=
40
, n
=
87 474)
71
Homocysteine
1.15 (1.09–1.22) (
N
=
20,
n
=
22 652) 72, 73
Coagulation marker
Fibrinogen
1.15 (1.13–1.17) (
N
=
40
, n
=
185 892)
66
Necrosis marker
Troponins
1.15 (1.04–1.27)
(
n
=
3 265)
58
Renal function marker
Urinary ACR
1.57 (1.26–1.95)
(
n
=
626)
74
Metabolic markers
HbA
1c
1.42 (1.16–1.74)
(
N
=
2,
n
=
2 442)
75
IGF-1
0.76 (0.56–1.04)
(
n
=
3 967)
76
Adiponectin
0.97 (0.86–1.09) (
N
=
14,
n
=
21 272)
77
Cortisol
1.10 (0.97–1.25)
(
n
=
2 512)
78, 79
BDNF
?
?
80–82
Insulin resistance
(HOMA)
1.46 (1.26–1.69) (
N
=
17,
n
=
51 161)
83
From: MMathews, L Liebenberg, E Mathews. How do high glycemic load
diets influence coronary heart disease?
Nutr Metab
2015;
12
(1): 6.
7
n
, number of participants;
N
, number of trials; CI, confidence interval; ACR,
albumin-to-creatinine ratio; Apo B, apolipoprotein-B; BDNF, brain-derived
neurotrophic factor; BNP, B-type natriuretic peptide; GDF-15, growth-
differentiation factor-15; HbA
1c
, glycated haemoglobin A
1c
; HDL, high-density
lipoprotein; HOMA, homeostasis model assessment; hsCRP, high-sensitivity
C-reactive protein; IGF-1, insulin-like growth factor-1; IL-6, interleukin-6;
LDL, low-density lipoprotein; MPO, myeloperoxidase; OPG, osteoprotegerin;
TNF-
α
, tumour necrosis factor-
α
.