
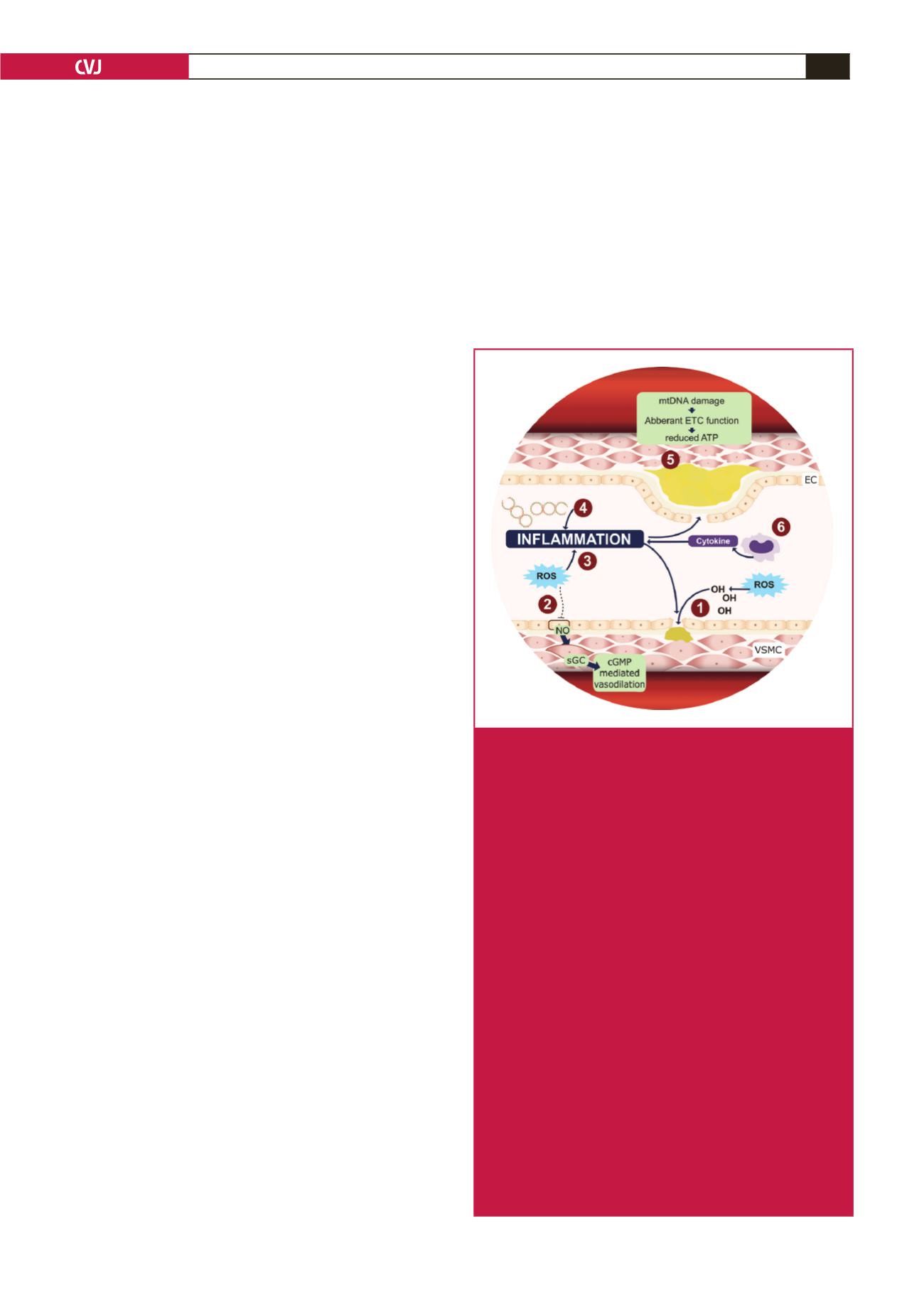
CARDIOVASCULAR JOURNAL OF AFRICA • Volume 29, No 2, March/April 2018
AFRICA
123
phosphorylation (OXPHOS) system (Fig. 1). In humans, mtDNA
contains 16 569 bps and is double stranded.
12
Depending on the
energy needs of a specific tissue, each cell can contain hundreds
to thousands of copies of mtDNA.
13
mtDNA is maternally
inherited and has a much higher mutation rate than nDNA,
possibly 10 to 17 times higher.
14
Maternal inheritance results in a
lack of bi-parental recombination and therefore the evolution of
mtDNA is defined by the emergence of distinct lineages called
haplogroups.
Multi-copy makes possible a condition called heteroplasmy,
where more than one genotype is present in the same cell/
tissue/organism; homoplasmy then, is where all mtDNA copies
carry the same allele. Notably, mtDNA is largely overlooked in
GWASs, and could possibly contribute to the missing heredity
of CVDs. Next we will consider two main arguments on the
possible role of mtDNA variants in CVDs.
Mitochondrial dysfunction and mtDNA damage in
vascular health
When considering mtDNA as a possible contributor to
the aetiology of CVD, it should also be considered from a
biological perspective. Much investigation has been conducted
in an attempt to elucidate the risk factors and physiological
mechanisms involved in the development of CVDs, such as
sub-clinical atherosclerosis, hypertension, cardiomyopathy and
type 2 diabetes.
15-20
An important common feature in all these conditions is
inflammation in some formor another (Fig. 2). This inflammatory
state is thought to be caused by oxidative stress, due to excessive
levels of reactive oxygen species (ROS). ROS can be produced
in several pathways, including by enzymes such as NADPH
oxidase, nitric oxide synthase, and enzyme complexes of the
electron transport chain (ETC).
21
The general mechanism of ROS involvement in CVDs is
ascribed to oxidative effects. For example, ROS contributes to
atherosclerotic lesion formation by oxidising lipids, promoting
vessel wall uptake of inflammatory cells, and enhancing
proliferation and hypertrophy of vascular smooth muscle cells
(VSMC).
21
Several studies have shown increased levels of ROS in
hypertensive humans and rats.
16,22,23
In cultured VSMCs for
example, ROS has been shown to cause changes in cellular
signalling pathways, favouring vasoconstriction.
15
A
mechanism for this could be that ROS reduces nitric oxide
(NO) bioavailability via quenching, impairing endothelium-
mediated vasodilation.
21,22,24
However, ROS along with other
factors of a dysfunctional mitochondrial energy metabolism (e.g.
nucleotides, Ca
2+
) also act as effectors of retrograde signalling
and the so-called cell danger response.
25-27
Mitochondria are considered the major producers of ROS
within the cell. In a recent article, Lopez-Armada
et al
.
18
reviewed
the role of mitochondrial dysfunction in the inflammatory
response and consequently in the pathology of various diseases,
including CVDs. The authors described how mitochondrial
dysfunction may modulate inflammatory processes by activating
redox-sensitive inflammatory pathways and the NLRP3
inflammasome. In the vasculature, these alterations lead to
disturbed endothelial homeostasis, which has been implicated
in the pathology of CVDs, such as atherosclerosis.
18
Indeed,
some improvements in disease presentation of hypertension
and diabetes have been observed in studies where chronic anti-
oxidant treatment is applied.
18,28,29
Another mechanism by which inflammation might be altered
by mitochondrial dysfunction is through the resultant release
of mtDNA into the cytosol and circulation. Because mtDNA
is similar to bacterial DNA and not methylated,
30
released
mtDNA molecules are thought to induce an inflammatory state,
which contributes to atherosclerosis and other inflammatory
diseases.
31-35
Fig. 2.
Mitochondrial dysfunction and mtDNA damage affect
vascular health in several ways. (1) ROS aids in lesion
formation by oxidising lipids, increasing the uptake of
inflammatory cells into the vascular wall and enhanc-
ing proliferation and hypertrophy in VSMC. (2) During
endothelium-dependant vasodilation, EC-released NO
activates sGC in VSMC to produce cGMP, signalling
a vasodilation response. ROS inhibits this mecha-
nism by quenching bioavailable NO molecules. (3)
Endothelial homeostasis is disturbed and plaque forma-
tion promoted when mitochondrial dysfunction leads to
ROS formation and activates redox-sensitive inflam-
matory pathways. (4) Circulating cell-free mtDNA is
similar in structure to bacterial DNA and invokes an
inflammatory response, contributing to atherosclerosis.
(5) Independent from ROS formation, mtDNA damage
leads to aberrant ETC function and reduced ATP
levels in VSMC. When cell viability is compromised,
apoptosis of VSMC occurs, accelerating plaque growth
and decreasing plaque integrity. (6) Through the same
mechanisms, apoptosis of monocytes occurs, releasing
inflammatory cytokines, contributing to inflammation
and consequently, increasing plaque formation and
vulnerability. ATP: adenosine triphosphate; cGMP: cyclic
guanosine monophosphate; EC: endothelial cell; ETC:
electron transport chain; NO: nitric oxide; ROS: reactive
oxygen species; sGC: soluble guanylyl cyclase; VSMC:
vascular smooth muscle cells.