
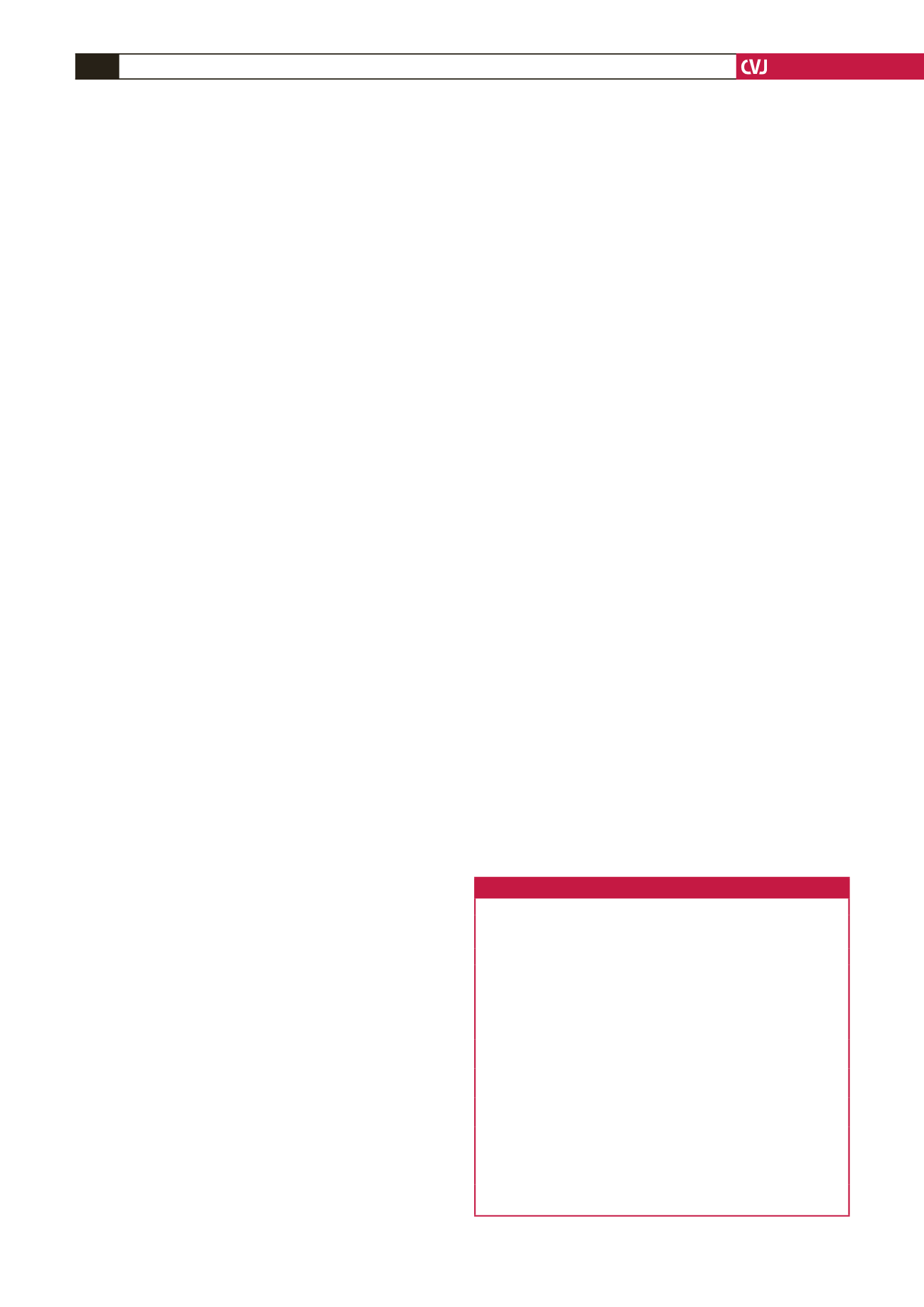
CARDIOVASCULAR JOURNAL OF AFRICA • Volume 29, No 2, March/April 2018
124
AFRICA
mtDNA damage has also been shown to promote
atherosclerosis directly, in the absence of oxidative stress. In a
study by Yu
et al
.,
36
VSMCs showed increased apoptosis and
decreased proliferation in a proof-reading deficient PolG
-/-
/
ApoE
-/-
mouse model. Increased secretion of pro-inflammatory
factors, tumour necrosis factor-
α
and interleukin-1
β
were also
reported and implicated in mtDNA release into the cytosol and
subsequent activation of the inflammasome.
The authors went on to test the applicability of their findings
in humans and concluded that an alternative mechanism
for mtDNA defects mediate atherosclerosis development,
independent of ROS; mtDNA defects lead to aberrant ECT
function and consequently reduce ATP content in VSMCs,
which then promote apoptosis and inhibit cell proliferation,
leading to increased atherosclerosis and risk of plaque rupture.
36,37
Plaque vulnerability is further promoted by mtDNA defects
via monocyte cell death and the resultant increased release of
inflammatory cytokine.
38
From these studies, it can be seen
that mitochondrial dysfunction, possibly as a result of mtDNA
variants or damage, can directly be implicated in mechanisms
that encumber vascular health.
mtDNA point mutations and cardiac involvement
Clinically proven mtDNA mutations are also an important cause
of inherited disease.
39
To date, more than 250 deleterious point
mutations and deletions of the mitochondrial genome have been
clinically proven to be associated with certain disease phenotypes
(www.mitomap.org). In several of these diseases, cardiovascular
symptoms are an important part of the aetiology.
Due to the very high levels of mtDNA population
variation seen, both within and between human populations,
the identification of mutations causing clinically manifesting
disease proves to be a challenge, despite the small size of the
mitochondrial chromosome. Initially, DiMauro and Schon had
set specific criteria for defining the pathogenicity of mtDNA
mutations.
40
The list has subsequently been updated to include
important methods such as functional testing and single-
fibre analysis, which can more specifically link genotype to
phenotype.
41,42
Notably, a pathogenicity scoring system for mitochondrial
tRNAs was devised by McFarland
et al
.
41
and further refined
by Yarham
et al
.
43
Mitchell
et al
.
44
also devised a pathogenicity
scoring system using variants in complex I mtDNA genes, but
this can be applied to any structural mtDNA mutation. A list of
these criteria is given in Table 1.
It should be noted that there are mtDNA mutations that are
exceptions to all the ‘rules’ in Table 1, and this was a critical
motivation for algorithms or clinical scoring systems to help
weigh the evidence that is presented for each mutation.
43,44
For a
clinically proven mutation to manifest as a diseased phenotype,
as in the case of primary mitochondrial disorders, the allele
frequency (heteroplasmy) needs to exceed a certain threshold,
usually above 60%, referred to as the phenotypical threshold
effect.
45
The biochemical threshold effect then refers to the ability of
the oxidative phosphorylation (OXPHOS) system to resist the
metabolic expression of deficiencies therein.
45,46
These deficiencies
may be caused by various factors involved in the expression and
regulation of the OXHPOS complexes.
There are many complexities to the expression of mtDNA
mutations; a classic example is the mitochondrial tRNAmutation
m.3243A
>
G, the most common of the mtDNA mutations
causing mitochondrial disease. The m.3243A
>
G mutation can
result in a vast array of clinical phenotypes affecting multiple
systems within the body, causing two distinct clinical syndromes:
maternally inherited diabetes and deafness (MIDD), and
mitochondrial encephalomyopathy, lactic acidosis, and stroke-
like episode (MELAS) syndrome in severe cases. Furthermore,
the age of onset of m.3243A
>
G-associated phenotypes spans
more than 50 years. The impact of several confounding factors,
including heteroplasmy levels, remains unclear.
47
Another group of well-studied mutations are those that
cause the disease Leber’s hereditary optic neuropathy (LHON).
In contrast to the m.3243A
>
G mutation, LHON has a tissue-
specific phenotype manifesting as bi-lateral blindness. Several
mtDNA mutations have been implicated in LHON, while
three of these mutations, namely m.3460G
>
A, m.11778G
>
A
and m.14484T
>
C located in subunits ND1, ND4 and ND6
of complex I, respectively, account for 90 to 95% of cases.
48
Unusually, these mutations can be detected as homoplasmic
variants without exerting a phenotype. Rather, disease penetrance
is significantly influenced by confounding factors such as
gender and environment (clinical penetrance is increased to
93% in smoking men),
49
and mtDNA haplogroup background
(haplogroup J, K and M7 increase risk of clinical penetrance).
50,51
The heart has especially high energy needs and relies heavily
on OXPHOS-derived ATP, such that one-third of cardiomyocyte
volume consists of mitochondria.
52
Not surprisingly then, the
myocardium is frequently affected in primary mitochondrial
disorders.
53
In a retrospective review study by Yaplito-Lee
et al
.,
54
33% of paediatric patients with definitive OXPHOS
disorders had cardiac manifestations. Several mtDNA mutations
(Fig. 3, Table 2 [online]) have also been shown to exhibit cardiac
involvement, either as part of a multi-system syndrome (most
frequently seen in MELAS), or as isolated occurrences, such as
in the absence of associated CVDs or risk factors thereof.
53,55,56
Hypertrophic cardiomyopathy (hCM) and pulmonary artery
hypertension (PAH) are the two phenotypes most commonly
seen as isolated cardiac manifestations of primary mitochondrial
Table 1. Criteria for defining the pathogenicity of mtDNA mutations
Criteria for pathogenicity of mtDNA mutations include
• The mutation must be present only in patients and not in controls
• The mutation must be present in varied mitochondrial genetic backgrounds
• The mutation must be the best mtDNA candidate variant to be pathogenic
• The mutation must affect functionally important domains
• Transfer of the mutated mtDNA to another cell line must be accompanied
by transfer of the cellular or molecular defect
• The mutation must not be a recognised, non-pathogenic, single-nucleotide
polymorphism
• The mutation must alter an area that is known to be highly conserved
throughout evolution
• The mutation must occur at varying levels within the cells (i.e. must be
heteroplasmic)
• A larger proportion of mutant mtDNA must correspond to a more severe
phenotype
• Single-fibre polymerase chain reaction must be performed by comparing
normal and abnormal fibres from muscle
• The secondary structure of the tRNA molecule must also be taken into
account when determining mt-tRNA mutation pathogenicity
These criteria need to be met in order for a mtDNA mutation to be classified as
‘disease causing’ for either structural or mt-tRNA mutations.
40-43