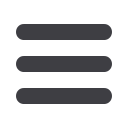
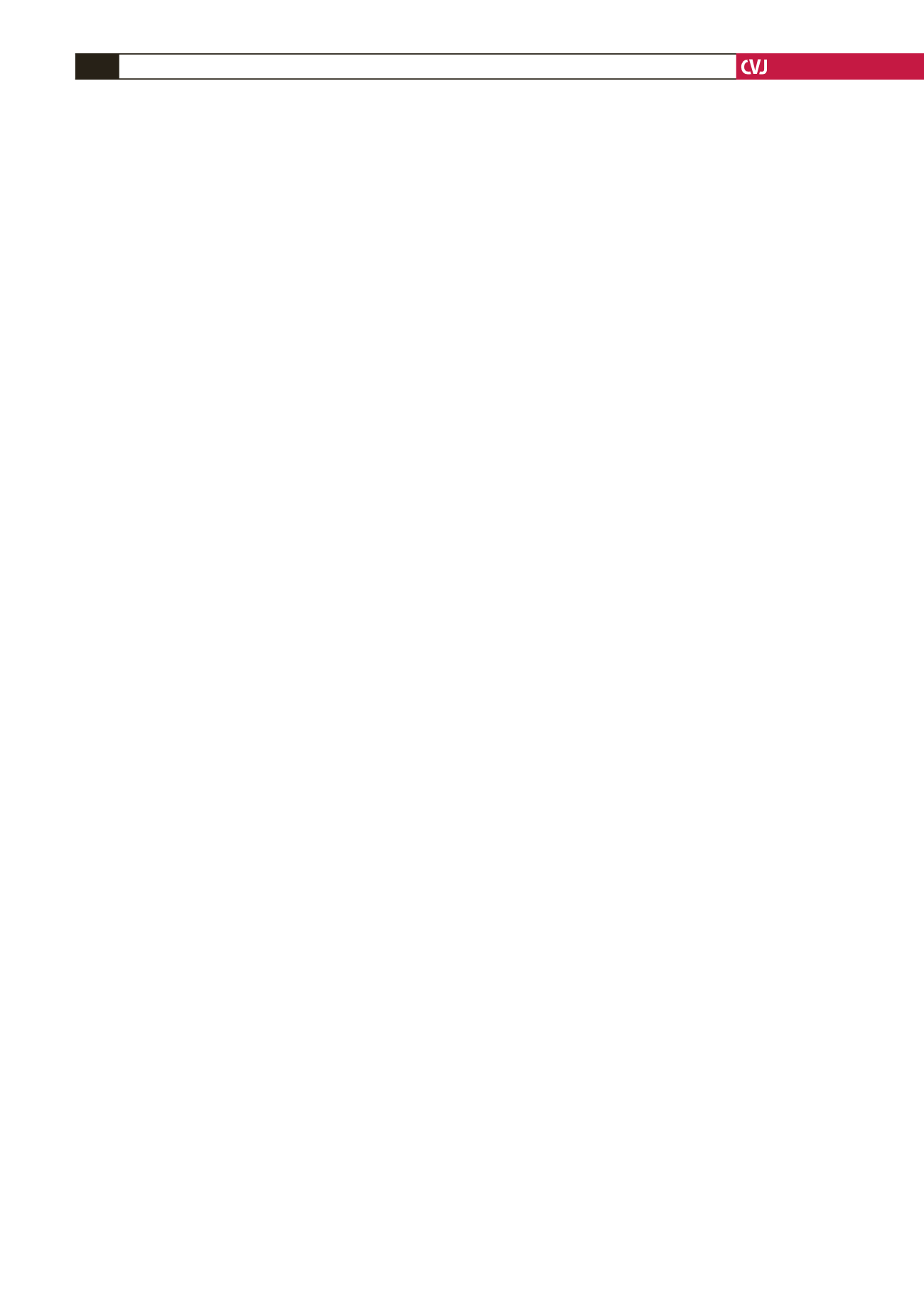
CARDIOVASCULAR JOURNAL OF AFRICA • Volume 29, No 1, January/February 2018
62
AFRICA
98. Lovelock JD, Baker AH, Gao F, et al. Heterogeneous effects of tissue
inhibitors of matrix metalloproteinases on cardiac fibroblasts.
Am J
Physiol Heart Circ Physiol
2005;
288
(2): H461–468.
99. Chakraborti S, Mandal M, Das S, Mandal A, Chakraborti T. Regulation
of matrix metalloproteinases: an overview.
Mol Cell Biochem
2003;
253
(1–2): 269–285.
100. Vincenti MP, Brinckerhoff CE. Signal transduction and cell-type specif-
ic regulation of matrix metalloproteinase gene expression: can MMPs be
good for you?
J Cell Physiol
2007;
213
(2): 355–364.
101. Klein T, Bischoff R. Physiology and pathophysiology of matrix metal-
loproteases.
Amino Acids
2011;
41
(2): 271–290.
102. Siwik DA, Chang DL, Colucci WS. Interleukin-1beta and tumor
necrosis factor-alpha decrease collagen synthesis and increase matrix
metalloproteinase activity in cardiac fibroblasts in vitro.
Circ Res
2000;
86
(12): 1259–1265.
103. MacKenna DA, Dolfi F, Vuori K, Ruoslahti E. Extracellular signal-
regulated kinase and c-Jun NH2-terminal kinase activation by mechani-
cal stretch is integrin-dependent and matrix-specific in rat cardiac
fibroblasts.
J Clin Invest
1998;
101
(2): 301–310.
104. MacKenna D, Summerour SR, Villarreal FJ. Role of mechanical factors
in modulating cardiac fibroblast function and extracellular matrix
synthesis.
Cardiovasc Res
2000;
46
(2): 257–263.
105. Manso AM, Elsherif L, Kang SM, Ross RS. Integrins, membrane-type
matrix metalloproteinases and ADAMs: potential implications for
cardiac remodeling.
Cardiovasc Res
2006;
69
(3): 574–584.
106. Ross RS. The extracellular connections: the role of integrins in myocar-
dial remodeling.
J Card Fail
2002;
8
(6 Suppl): S326–331.
107. Coker ML, Jolly JR, Joffs C,
et al
. Matrix metalloproteinase expression
and activity in isolated myocytes after neurohormonal stimulation.
Am
J Physiol Heart Circ Physiol
2001;
281
(2): H543–551.
108. Sabri A, Rafiq K, Seqqat R, Kolpakov MA, Dillon R, Dell’italia LJ.
Sympathetic activation causes focal adhesion signaling alteration in
early compensated volume overload attributable to isolated mitral regur-
gitation in the dog.
Circ Res
2008;
102
(9): 1127–1136.
109. Ogawa K, Chen F, Kuang C, Chen Y. Suppression of matrix metallopro-
teinase-9 transcription by transforming growth factor-beta is mediated
by a nuclear factor-kappaB site.
Biochem J
2004;
381
(Pt 2): 413–422.
110. Rouet-Benzineb P, Gontero B, Dreyfus P, Lafuma C. Angiotensin II
induces nuclear factor- kappa B activation in cultured neonatal rat
cardiomyocytes through protein kinase C signaling pathway.
J Mol Cell
Cardiol
2000;
32
(10): 1767–1778.
111. Seeland U, Haeuseler C, Hinrichs R,
et al
. Myocardial fibrosis in
transforming growth factor-beta(1) (TGF-beta(1)) transgenic mice is
associated with inhibition of interstitial collagenase.
Eur J Clin Invest
2002;
32
(5): 295–303.
112. Bujak M, Frangogiannis NG. The role of TGF-beta signaling in
myocardial infarction and cardiac remodeling.
Cardiovasc Res
2007;
74
(2): 184–195.
113. Zheng J, Chen Y, Pat B,
et al
. Microarray identifies extensive downregu-
lation of noncollagen extracellular matrix and profibrotic growth factor
genes in chronic isolated mitral regurgitation in the dog.
Circulation
2009;
119
(15): 2086–2095.
114. Zhang YM, Bo J, Taffet GE,
et al
. Targeted deletion of ROCK1 protects
the heart against pressure overload by inhibiting reactive fibrosis.
FASEB J
2006;
20
(7): 916–125.
115. Jellis C, Martin J, Narula J, Marwick TH. Assessment of nonischemic
myocardial fibrosis.
J Am Coll Cardiol
2010;
56
(2): 89–97.
116. Dell’Italia LJ, Meng QC, Balcells E,
et al
. Increased ACE and chymase-
like activity in cardiac tissue of dogs with chronic mitral regurgitation.
Am J Physiol
1995;
269
(6 Pt 2): H2065–2073.
117. Stewart JA, Jr., Wei CC, Brower GL,
et al
. Cardiac mast cell- and
chymase-mediated matrix metalloproteinase activity and left ventricular
remodeling in mitral regurgitation in the dog.
J Mol Cell Cardiol
2003;
35
(3): 311–319.
118. Tallaj J, Wei CC, Hankes GH,
et al
. Beta1-adrenergic receptor blockade
attenuates angiotensin II-mediated catecholamine release into the cardi-
ac interstitium in mitral regurgitation.
Circulation
2003;
108
(2): 225–230.
119. Lin A, Stewart R. Medical treatment of asymptomatic chronic aortic
regurgitation.
Expert Rev Cardiovasc Ther
2011;
9
(9): 1249–1254.
120. Weidemann F, Herrmann S, Stork S,
et al
. Impact of myocardial fibrosis
in patients with symptomatic severe aortic stenosis.
Circulation
2009;
120
(7): 577–584.
121. Weinberg EO, Schoen FJ, George D,
et al
. Angiotensin-converting
enzyme inhibition prolongs survival and modifies the transition to heart
failure in rats with pressure overload hypertrophy due to ascending
aortic stenosis.
Circulation
1994;
90
(3): 1410–1422.
122. Perry GJ, Wei CC, Hankes GH,
et al
. Angiotensin II receptor blockade
does not improve left ventricular function and remodeling in suba-
cute mitral regurgitation in the dog.
J Am Coll Cardiol
2002;
39
(8):
1374–1379.
123. Babbitt CJ, Shai SY, Harpf AE, Pham CG, Ross RS. Modulation of
integrins and integrin signaling molecules in the pressure-loaded murine
ventricle.
Histochem Cell Biol
2002;
118
(6): 431–439.
124. Bloor CM, Nimmo L, McKirnan MD, Zhang Y, White FC. Increased
gene expression of plasminogen activators and inhibitors in left ventric-
ular hypertrophy.
Mol Cell Biochem
1997;
176
(1–2): 265–71.
125. Brower GL, Chancey AL, Thanigaraj S, Matsubara BB, Janicki JS.
Cause and effect relationship between myocardial mast cell number and
matrix metalloproteinase activity.
Am J Physiol Heart Circ Physiol
2002;
283
(2): H518–525.
126. Janicki JS, Brower GL, Gardner JD,
et al.
Cardiac mast cell regulation
of matrix metalloproteinase-related ventricular remodeling in chronic
pressure or volume overload.
Cardiovasc Res
2006;
69
(3): 657–665.
127. Nagatomo Y, Carabello BA, Coker ML,
et al
. Differential effects of
pressure or volume overload on myocardial MMP levels and inhibitory
control.
Am J Physiol Heart Circ Physiol
2000;
278
(1): H151–161.
128. Chancey AL, Brower GL, Peterson JT, Janicki JS. Effects of matrix
metalloproteinase inhibition on ventricular remodeling due to volume
overload.
Circulation
2002;
105
(16): 1983–1988.
129. Ljungvall I, Rajamaki MM, Crosara S,
et al.
Evaluation of plasma
activity of matrix metalloproteinase-2 and -9 in dogs with myxomatous
mitral valve disease.
Am J Vet Res
2011;
72
(8): 1022–1028.
130. Iwanaga Y, Aoyama T, Kihara Y, Onozawa Y, Yoneda T, Sasayama S.
Excessive activation of matrix metalloproteinases coincides with left
ventricular remodeling during transition from hypertrophy to heart
failure in hypertensive rats.
J Am Coll Cardiol
2002;
39
(8): 1384–1391.
131. Moustafa SE, Kansal M, Alharthi M, Deng Y, Chandrasekaran K,
Mookadam F. Prediction of incipient left ventricular dysfunction in
patients with chronic primary mitral regurgitation: a velocity vector
imaging study.
EurJ Echocardiogr
2011;
12
(4): 291–298.
132. Magne J, Mahjoub H, Dulgheru R, Pibarot P, Pierard LA, Lancellotti
P. Left ventricular contractile reserve in asymptomatic primary mitral
regurgitation.
Eur Heart J
2014;
35
(24): 1608–1616.
133. Le Tourneau T, de Groote P, Millaire A,
et al.
Effect of mitral valve
surgery on exercise capacity, ventricular ejection fraction and neurohor-
monal activation in patients with severe mitral regurgitation.
J Am Coll
Cardiol
2000;
36
(7): 2263–2269.
134. Mehta RH, Supiano MA, Oral H,
et al
. Compared with control
subjects, the systemic sympathetic nervous system is activated in patients
with mitral regurgitation.
Am Heart J
2003;
145
(6): 1078–1085.